Study and Research Group on Microwaves (GERM)
Transversal action created at the initiative of D. Stuerga (CNU 31), the Study and Research Group on Microwaves (GERM) was recognized as a research team in 2002.
The wish was to develop a team dedicated to the control and use of the electromagnetic energy vector more specifically microwave or microwave in instrumentation and sensor as well as in chemistry and processes.
Didier STUERGA
Head
didier.stuerga[at]u-bourgogne.fr
Phone: +33 (0)3 80 39 61 82
Members
The primary vocation of the Study and Research Group on Microwaves (GERM) is the reasoned mastery of electromagnetic energy in microwave or microwave bands. This mastery involves the development of broadband metrology tools (MHz to GHz) and high power applicators (several kW) in an engineering logic in sensors, reactors, processes for the development and formulation of materials and nanomaterials The first objective is to design and develop high performance microwave applicators and reactors. These reactors are tools for the development and development of selective microwave processes for the development of materials and nanomaterials. These processes are of the one step soft solution process type. The environmental objective is to reduce separation and isolation procedures that are costly in energy and generate polluting effluents, the treatment and recycling of which no longer make profitable old processes. The second objective is the design and development of techniques original microwave transduction. The combination of electromagnetic calculation tools and broadband dielectric characterizations allows the development of sensors and transducers. The applications are multiple since they range from the detection of gases and pollutants to the detection of damage on polymer materials (collaboration with ICMUB and LASMEA Clermont-Ferrand). The new fields of application relate to the development of microwave catheters (Dijon University Hospital) and a microwave microscopy technique.
Thematics
The diagram in Figure 1 describes the overlapping of the different lines of research. The electromagnetic calculation tools (Axis 1) associated with the dielectric characterization tools (Axis 2) allow the control of the microwave energy vector. The objective is to understand and master the electromagnetic distributions in cavities or waveguides charged by dissipative dielectrics.
Technological transfer
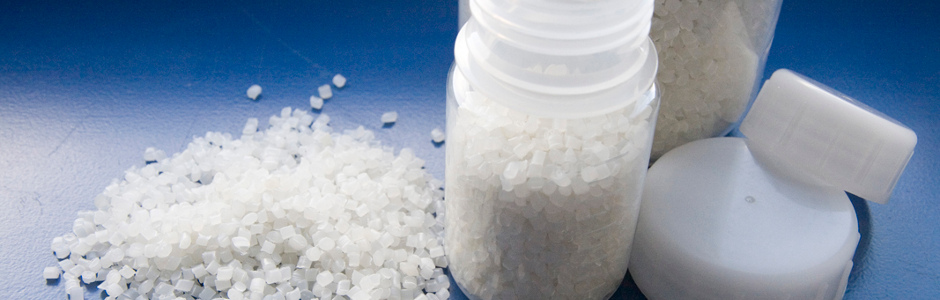
One of the specificities of this research group is the Naxagoras Technology promotion project. This project has been carried out since July 2007 by a company called Naxagoras Technology. Since July 2007, D. Stuerga has enjoyed the status of scientific and technical competition, as defined by article 25-2 of law n ° 99-587 of July 12, 1999 on innovation and research (article L413-8 to L413-11 of the research code, positive decision of the ethics committee in June 2007, authorization of scientific and technical assistance on July 16, 2007 and development agreement n ° 2007-0373 of September 11, 2007). Naxagoras Technology benefits from Research Tax Credit (CIR) approval and the tax status of a young innovative company. The partnership between the GERM team and Naxagoras Technology marks a new stage in the development of GERM.
As part of the partnership with the startup Naxagoras Technology, D. Stuerga is participating in several projects associating SMEs in the plastics industries (compounders, formulators and processors) and paints and coatings. These research and development projects implying a consistent conceptual and scientific approach, they are supported by OSEO aids. The objectives are the analysis and study of mechanical and photocatalytic functionalities of polymeric coatings with the aim of developing self-cleaning coatings. A business cluster around nanoformulation for the plastics industry has been set up in the Burgundy region by the Alize Plasturgie Bourgogne (PlastiNano Bourgogne) structure.
Over the 2007-2011 period, D. Stuerga participated in the European IDEAL-Cell contract (Innovative Dual mEmbrAne fueL Cell, www.ideal-cell.eu). The aim of the intervention was to develop materials of the proton conductor type in nanometric form. This project brought together 9 European partners for an amount of 4 MEuros. The ultimate goal of this project was the development of a new type of double membrane fuel cell.
Over the period 2012-2015, D. Stuerga intervenes under the same conditions in an ANR-LIMA industrial research project (Light Interactions Materials Aspect, www.pole-moveo.org/pdf-projets-das/Lima-A. pdf).). This project associates 6 partners including the Ecole des Mines de Paris and the PSA group for an amount of 2 MEuros. This project aims to develop model nanometric pigments for goniochromatic paints. Finally, a study contract with Naxagoras Technology as co-financier (MicroNano 3, SMT6-ONOV 2012, 20 KE) will allow testing of a new version of reactor in 2013.
- kc_data:
- a:8:{i:0;s:0:"";s:4:"mode";s:0:"";s:3:"css";s:0:"";s:9:"max_width";s:0:"";s:7:"classes";s:0:"";s:9:"thumbnail";s:0:"";s:9:"collapsed";s:0:"";s:9:"optimized";s:0:"";}
- kc_raw_content:
- [kc_row use_container="yes" _id="49722"][kc_column width="60.23%" _id="850187"][kc_column_text _id="756634"]
Study and Research Group on Microwaves (GERM)
Transversal action created at the initiative of D. Stuerga (CNU 31), the Study and Research Group on Microwaves (GERM) was recognized as a research team in 2002. The wish was to develop a team dedicated to the control and use of the electromagnetic energy vector more specifically microwave or microwave in instrumentation and sensor as well as in chemistry and processes. [/kc_column_text][/kc_column][kc_column width="39.76%" _id="913543"][kc_column_text _id="741834"]Didier STUERGA
Head
didier.stuerga[at]u-bourgogne.fr Phone: +33 (0)3 80 39 61 82
[/kc_column_text][/kc_column][/kc_row][kc_row use_container="yes" _id="945343"][kc_column width="12/12" video_mute="no" _id="846307"][kc_spacing height="30px" _id="609107"][/kc_column][/kc_row][kc_row use_container="yes" _id="586812" cols_gap="{`kc-css`:{}}" force="__empty__" row_class="full bg-rs" animate="fadeInLeft||"][kc_column width="2%" video_mute="no" _id="397522"][/kc_column][kc_column width="30%" video_mute="no" _id="551088"][kc_spacing height="20" _id="711971"][kc_column_text _id="561019"]Members
[/kc_column_text][kc_spacing height="20" _id="502875"][kc_column_text _id="63551"] {slide=Permanents}- Pr. Didier STUERGA (CNU 31)
- Pr. Pierre PRIBETICH (CNU63)
- Jérôme ROSSIGNOL (HDR CNU 63)
Thematics
The diagram in Figure 1 describes the overlapping of the different lines of research. The electromagnetic calculation tools (Axis 1) associated with the dielectric characterization tools (Axis 2) allow the control of the microwave energy vector. The objective is to understand and master the electromagnetic distributions in cavities or waveguides charged by dissipative dielectrics. [/kc_column_text][kc_spacing height="20" _id="918692"][kc_single_image image_size="full" _id="316715" image_source="media_library" image="12675" on_click_action="lightbox"][kc_column_text _id="590630"] {slide=More informations} These first two lines of research involve theoretical approaches combining modeling, numerical simulation, original experimental protocols as well as high-level microwave instrumentation and metrology. These tools are used to design and optimize microwave laboratory reactors and pilot installations (Axis 3). The dielectric characterization tools (Axis 2) now allow the development of a complete detection chain associating sensors, direct interrogation circuitry or distance. A multi-sensor-multitransduction bench type installation allows comparative studies both between sensors and between desired species. Axis 4 develops original nanomaterials in the form of complex oxides and metals in powder form, in soils and colloidal suspensions. The development of processes involves taking into account the coupling between electromagnetism, hydrodynamics, thermal and physico-chemistry. The latter including the control of species in solutions and the nucleation, growth, agglomeration and aggregation processes. Finally, the transposition to the kilogram scale is developed in close collaboration with a private structure developing and operating a production pilot, owned by the team. Finally, Axis 5 aims to formulate and integrate these nanomaterials in diverse and varied environments. The formulation is understood in a broad sense by studying completely original and innovative electromagnetic procedures. The skills developed in the Thematic Axes are put into practice in the projects described on the right side of Figure 1. {/slide} [/kc_column_text][/kc_column][kc_column width="2%" _id="205229"][/kc_column][kc_column width="49%" _id="676316"][kc_spacing height="60px" _id="286126"][kc_column_text _id="58659"] {slide=Axis1 : Modeling}general context
The research activity concerns the modeling and simulation of electromagnetic phenomena, specifically for dielectric media with high dielectric losses. The objective is the analysis and forecasting of the topology of electromagnetic fields specific to environments with high dielectric losses. The strong point is the development of calculation tools for the design of high performance microwave applicators and planar circuits.our problematic :
Simulation or Modeling: A microwave reactor includes a coupling device (waveguide) with the microwave source. The reactor consists of several more or less dissipative materials on the electromagnetic level. The objective is the focusing of energy at the level of the reactive medium. To achieve this objective, it is necessary to know the distributions of electromagnetic fields within the entire system (coupling device and reactor). One possible approach is digital simulation, which provides access to the spatial distributions of electromagnetic fields within such structures. However, such an approach, even if it is predictive, does not necessarily make it possible to grasp the mechanisms that control the evolution of the field distributions within the structure studied. Within a propagative structure, it is well known that there are particular distributions called eigen modes of the structure. According to the dimensions and the types of structure, we will be able to have coexistence of several eigen modes (multimodal structure). In such structures, numerical simulation can only predict the result of this mixture. The analysis in eigen modes is a necessity for the interpretation of the numerical simulation, and therefore the optimization of the structure insofar as the objective is to control the spatial distribution of the electromagnetic energy in relation to the heat treatment wish. The interest and the effectiveness of the coupling digital simulation-analysis in eigen modes then appear in an obvious way. A digital simulation alone will be similar to a digital experiment which is limited to predicting the distribution of fields corresponding to a given configuration. The analysis in eigen modes only specifies the conditions of existence of the different modes likely to exist, and therefore the global spatial distribution of electromagnetic fields.OWN MODES ANALYSIS:
The eigenmode analysis technique developed combines an analytical and numerical approach. The analytical step makes it possible to postulate the expression of the components of electromagnetic fields. The connection of tangential components at the interfaces leads to a linear system (matrix). The numerical step based on the properties of the functions of complex variables (residue theorem and contour integral) makes it possible to obtain both the field components and the values of the different propagation constants. This analysis method developed in rectangular and cylindrical geometry makes it possible to obtain all of the modes without any a priori on their nature (LES and LSM modes for example).NUMERICAL SIMULATION :
The finite difference method in the frequency domain (FDFD) is used. The chosen resolution scheme is original in that it replaces the classical iterative schemes (Gauss-Seidel, Choleski) with a direct matrix inversion method associated with the discretized system. The reasons for this choice result from the stability and consistency criteria of the numerical scheme (truncation error). This calculation code enables electromagnetic field distributions to be obtained in record time for practical reactor-type configurations.THE FRACTAL PLANAR ANTENNAS:
The double need for high transmission rates and physical grouping of antennas requires the development of new antenna typologies. Planar antennas have proven their effectiveness in meeting this expectation. For several years, research on new forms of planar antennas, composed from original fractal patterns not offered, has been initiated by P. Pribetich. The first published works (Pribetich et al, A new planar microstrip resonators for microwave circuits: the quasi-fractal microstrip resonator, Microwave and optical technology letters, May 1999) are cited several times as a reference in many international patents (Antennae, US Patent 7015868, 2006; Multifrequency microstrip patch antenna with parasitic coupled elements, US Patent 7202818, 2007; Loaded antenna US Patent 7312762, 2007; Multilevel Broadside high-directivity microstrip patch antennas, US Patent 7423593, 2008). We are continuing this research in order to identify and refine the concepts that would allow us to perfectly master the effects of this fractal division, with a view to widening the field of application of this original form to other circuits and microwave functions such as filters. planar or even new nanocircuits for nanoelectronics.{/slide}
[/kc_column_text][kc_column_text _id="518657"] {slide=Axis 2 : CARPEDIEM}Characterization of the Electrical Properties of Materials (J. Rossignol)
Initiated by the GERM group in 2005, broadband microwave transduction (MHz to GHz) appears to be a relevant transduction technique because it operates at room temperature, at low energy cost and low manufacturing cost. The objective is the design and manufacture of the sensors and the measurement chain. One of the original features of this project is the specific development aspect of innovative sensitive materials. A modeling aspect is developed in parallel (FDTD, HFSS). The long-term strategy is to develop a multi-sensor lab-on chip. With expertise in terms of radio frequencies and microwaves, wireless technology is seen to be reinforced in the sensor activity. The CARPEDIEM project benefits from the support of the ANR CAPBTX. In 2012, this ANR guaranteed PostDoc status for B. de Fonseca. The CARPEDIEM project has undergone numerous internships (M2) and three doctoral theses, two of which are in progress. This project is declined in various fields of application which are described below.NOSE (NanOSniffers Electromagnétiques)
The results of the first thesis (Julien Jouhannaud, 2008) relating to this thematic made it possible to define an original protocol for gas detection by microwave broadband measurements. This microwave transduction, unlike conventional conductimetric transduction methods, leads to an interaction spectrum between the sensitive material and the surrounding gas, unlike conventional sensors providing current or impedance type information. This microwave method uses the dielectric and conductimetric response of a material in the presence of a gas. The current objective is to study the influence of humidity on the detection of atmospheric pollutants by microwave transduction. The sensitive materials used are of two types, molecular compounds (ICMUB with M. Bouvet and LASMEA with M. Pauly and Brunet) and metallic semiconductors (ICB). A thesis is underway (Bouvet-Rossignol co-supervision, 2009-2012). The subject of M. G. Barochi's thesis is the development and optimization of gas sensors whose sensitive element is a deposit of cobalt phthalocyanine (PcCo). The defense is scheduled for early 2013.DEMACO (Detection of damage in COmposite materials)
The result of a lasting collaboration with the Materials Center of the Ecole des Mines de Paris (Pr. Thionnet), a technique for detecting damage in composite materials was created in 2007. Two contracts with the company GDF-SUEZ allowed the study of damage to composite tanks for CNG (Gaz Natural Vehicle).CATHARE: (RADIO FREQUENCY ANALYSIS CATHETER)
The objective is the development of an original microwave catheter. This device aims to detect and characterize burns caused by certain treatments of cardiac arrhythmia. As part of a Young Entrepreneur Researcher thesis, Mr Brusson started his thesis in 2010 under the supervision of J Rossignol and co-supervised by M. Binzack (LE2I) and M. G. Laurent cardiologist (CHU)MICROWAVE MICROSCOPY
After a first macroscopic homemade prototype in 2005 (Master SEA), GERM developed a second microwave microscope device. Subsequently, collaboration with the OSNC team from 2009 to 2012, led to a regional funding project for a non-scalable SMM commercial device (PARI-SMT5 project) for metallurgy applications. The GERM for its part has developed a multi-scale and multi-measure device for research activities and for the industry in the fields of electronics and materials (AES 2016 guest conference). This variation of CARPEDIEM is currently reflected in a publication on ACS.RECTENNA (RECtified AnTENNA, P. Pribetich
The skills in materials and antenna synthesis with a very high quality factor allow the implementation of an energy recovery project in the microwave fields in the form of so-called rectified antenna. Three students are working on this subject (PFE internship, Esirem). {/slide} [/kc_column_text][kc_column_text _id="392674"] {slide=Axis 3 : Microwave reactors}General context
The latest developments in our calculation tools now allow us to model and simulate three-dimensional industrial microwave applicators. The original character of our calculation codes is to take into account a priori and not a posteriori the existence of high dielectric losses. These calculation tools have made it possible to develop and optimize a Microclave Autoclave Reactor (RAMO) now used routinely in the Laboratory for the development of nanomaterials. The reactor itself is of cylindrical geometry and made up of several dissipative materials. Figure 1 describes the device. [caption id="" align="aligncenter" width="216"]FIg.1 Le dispositif[/caption] The objective is the focusing of energy at the level of the reactive medium in order to ensure a volume nucleation of the nanoparticles produced. To achieve such an objective, it is necessary to know the electromagnetic field distributions within the entire system (coupling device and reactor itself). The final objective of this work was to define the operating conditions ensuring the highest levels of focus of the electric field within the reactor.
Problematic :
What is the consequence in terms of modal analysis (TE, TM and HEM) of the strong dependence on temperature of the dielectric properties of the media? Can we envisage couplings of the modes between them? To what extent do high levels of focus of electric fields modify the associated heating curves? Are there possibilities for thermal bistability? Consequence in terms of thermal gradients and nucleation of nanoparticles in the heated medium?CONCEPT OF THERMAL TRAJECTORIES:
The three categories of modes of this structure have been identified: Electric Transverses (TE), Magnetic Transverses (TM) and Hybrids (HEMn). The strong thermal dependence of the dielectric properties induces a dynamic in the complex plane resulting in thermal trajectories in the form of loops. The first historical example of a loop is described in Figure 2 for a dissipative log and a waveguide having radii of 20 mm and 102.5 mm respectively.MODEL COUPLING:
Depending on the size of the log and in relation to the thermal dependence of the dielectric properties, the different thermal trajectories move and deform in the complex plane, possibly causing "couplings" between the viable modes of the structure. [caption id="" align="aligncenter" width="240"]FIg.2 Trajectoire thermique du mode TE01[/caption] Each of the types of modes has an optimal focus for a precise temperature. During heating and due to the coupling, an energy relay between the modes will operate. The analysis of the radial profiles clearly highlighted the role played by a third dielectric medium on the extent of the focusing within the dissipative log.
Electrothermal coupling:
The study of the electrothermal coupling clearly illustrates the influence of the evolution with the temperature of the dielectric losses in term of heating dynamics. Indeed, this work has shown that despite dielectric losses for water which decrease continuously with temperature, one can observe an inflection point on the heating curves. The focusing phenomenon within the dielectric log largely compensates for the drop in the capacity of water to convert electromagnetic energy into heat at 2.45 Ghz. These thermal runaway have been interpreted in terms of a jump between two stable branches (thermal bistability). This phenomenon appears to be detrimental to the control of the heating curves. Indeed, we have shown that for a radius of 20 mm, the temperature stabilizes at 40 ° C and does not vary any more, whereas for a radius of 40 mm, there appears a thermal runaway with a slope parallel to the axis of the ordinates for the heating curve. The practical consequences can be dramatic.Microwave Reactors and Processes:
Thermal gradients and Nucleation: The evolution of the couplings between the modes during heating should be able to ensure a relative uniformity of the energy dissipated during heating. The advantage of the most uniform possible heating induced by the distribution of dissipated energy is of first order for thermally activated nucleation reactions (NAT). Indeed, under such operating conditions, the priming by volume of the reaction must make it possible to decouple nucleation and growth, in particular to control the reseeding of the solution. This is in contrast to the operating conditions of conventional heating, where the parietal thermal gradients between the surface and the reactor core predominantly cause surface nucleation followed by diffusion of the entities in the solution. The mode of operation of the microwave reactor, insofar as it may favor volume nucleation, therefore appears to be completely different from conventional reactors. {/slide} [/kc_column_text][kc_column_text _id="437325"] {slide=Axis 4 : Nanomaterials}General context
The MITE-Flash process is the third generation of nanomaterials development process developed by D. Stuerga. The acronym MIT stands for Microwave Induced Thermohydrolysis. If all three generations are based on the specifics of microwave heating, the chemical operating conditions are relatively different. The first two generations are thermohydrolysis while the third generation can be described as thermoethanolysis.Generation 1 :
The first generation uses thermohydrolysis in aqueous solution. The initial solutions are obtained by dissolving the metal salt in an acidic aqueous solution. The heating of these solutions makes it possible to directly obtain oxide nanoparticles in a single step according to the thermohydrolysis type reaction scheme. Depending on the metals selected, nitrates, chlorides or sulfates are used as precursors. Nitrates are the most advantageous in terms of solubility and aggressiveness of solutions vis-à-vis metal parts. The use of hydrated salts is not a problem. The possibilities are reduced since the first major drawback is the impossibility of preparing oxides of complex compositions. The second major drawback is the low yields and the aggressiveness of the solutions with respect to metals, particularly in the case of the use of chloride. The initial solutions are acidic (pH <1) and become so the more so as the duration of the microwave treatment is important. This gives aqueous solutions of hydrochloric or nitric acid several times molar. The third drawback is the limited extension of the monodispersity and monocrystallinity zone (restricted range of concentration of precursors and of pH). Finally, the fourth drawback is the very asserted ionic character of the solutions which induce very high electrical conductivity levels. This is highly detrimental to microwave processing since the dielectric losses of the solutions are very high and complicate the optimized application of microwave energy on the reactors.Generation 2 :
This second synthetic route makes it possible to obtain simple or complex metal oxides in the form of monodispersed nanocrystals in sizes. The initial solutions are obtained by dissolving the metal salt (s) in ethanol in the presence of sodium ethanolate. This mixture causes the preliminary reaction to prepare the ethanolate (s) of the metal salts dissolved. This preliminary or first step reaction also produces sodium chloride and sodium hydroxide. Depending on the metals selected, nitrates, chlorides or sulfates are used as precursors. Heating the mixture allows direct obtaining of simple or complex oxide particles. The third step consists of multiple washes with ethanol and water which are necessary to remove the sodium chloride and sodium hydroxide resulting from the synthesis reactions. The first major drawback is the first step. It corresponds to the preparation of the precursor. In addition to its implementation which complicates the process, it generates in the reaction medium large amounts of sodium chloride and sodium hydroxide. These products require numerous washes of the powders in order to guarantee their total elimination of these parasitic products. This washing or isolation phase can be considered as a third step in its own right which appears to be excessively costly in terms of energy and time. In addition, the majority of metal salts are hydrated. For these, we must therefore further increase the amount of sodium ethanolate, proportionally increasing the amount of sodium hydroxide produced. These numerous washes result in a loss of the oxide produced. Precipitation yields taking into account the isolation step are therefore relatively low (<50%). The second disadvantage is that many metal salts exist only in the form of chlorides which often pose problems of solubility in ethanol. In this case, the preparation of the precursor appears to be delicate or even impossible. It leads to very low yields. The third drawback follows directly from the previous one. Indeed, the presence of sodium chloride and or metal chlorides in the final solutions is highly detrimental to the metal parts. The fourth drawback is identical to that of the first generation. The ionic nature of the solutions induces very high electrical conductivity levels which are highly detrimental to microwave treatment.Generation 3 :
This third generation, developed from 2005, makes it possible to develop clusters like nanomaterials without the need to wash the medium (integral one step). Polyoxometallate (POM) or more specifically Nano Building Blocks (NBB) types are produced. The size range of elementary particles is located between the tens of Angstroms and a few tens of nanometers. These particles can associate with each other spontaneously or in a controlled manner by adding additives such as surfactants. These can promote the solubilization of the initial precursors and considerably increase the stability of the suspensions produced. This process can be defined by flash thermoethanolysis induced by microwave heating or (MITE-Flash or Microwave Induced ThermoEthanolysis Flash). The main advantages and advantages of this process appear at seven different levels relating on the one hand to the products produced: composition, specific surface and average size, nature of the products produced, and on the other hand to the implementation of the process: simplicity , nature of the precursors and the initiation, This latest generation has been the subject of a patent. The first advantage of our one-step process is that it responds to environmental issues of cost reduction and pollutant releases. In fact, the process generates practically no secondary or parasitic product involving costly purification and / or recycling procedures. The second advantage of our process relates to the safety and toxicological aspect of nanomaterials. Indeed, the possible toxicity of the latter, particularly in powdered forms, remains an open question to this day. The advantage of our process is to produce suspensions, pastes or slip directly usable in a single step (one-step). The handling and possible dispersion of the nanoparticles are therefore reduced to the strict minimum guaranteeing high process security. These two assets confer a high environmental quality character to our family of production process which clearly falls under the "Green Chemistry". {/slide} [/kc_column_text][/kc_column][/kc_row][kc_row use_container="yes" _id="254017"][kc_column width="12/12" video_mute="no" _id="964602"][kc_spacing height="50px" _id="141261"][/kc_column][/kc_row][kc_row use_container="yes" _id="506085" cols_gap="{`kc-css`:{}}" force="__empty__" animate="fadeInUp||"][kc_column width="20%" _id="451369"][/kc_column][kc_column width="60%" _id="224452"][kc_column_text _id="87258"]Technological transfer
[/kc_column_text][kc_spacing height="20" _id="442820"][kc_single_image image_size="full" _id="616023" image_source="media_library" image="12707"][kc_column_text _id="810364"]One of the specificities of this research group is the Naxagoras Technology promotion project. This project has been carried out since July 2007 by a company called Naxagoras Technology. Since July 2007, D. Stuerga has enjoyed the status of scientific and technical competition, as defined by article 25-2 of law n ° 99-587 of July 12, 1999 on innovation and research (article L413-8 to L413-11 of the research code, positive decision of the ethics committee in June 2007, authorization of scientific and technical assistance on July 16, 2007 and development agreement n ° 2007-0373 of September 11, 2007). Naxagoras Technology benefits from Research Tax Credit (CIR) approval and the tax status of a young innovative company. The partnership between the GERM team and Naxagoras Technology marks a new stage in the development of GERM.
As part of the partnership with the startup Naxagoras Technology, D. Stuerga is participating in several projects associating SMEs in the plastics industries (compounders, formulators and processors) and paints and coatings. These research and development projects implying a consistent conceptual and scientific approach, they are supported by OSEO aids. The objectives are the analysis and study of mechanical and photocatalytic functionalities of polymeric coatings with the aim of developing self-cleaning coatings. A business cluster around nanoformulation for the plastics industry has been set up in the Burgundy region by the Alize Plasturgie Bourgogne (PlastiNano Bourgogne) structure. Over the 2007-2011 period, D. Stuerga participated in the European IDEAL-Cell contract (Innovative Dual mEmbrAne fueL Cell, www.ideal-cell.eu). The aim of the intervention was to develop materials of the proton conductor type in nanometric form. This project brought together 9 European partners for an amount of 4 MEuros. The ultimate goal of this project was the development of a new type of double membrane fuel cell. Over the period 2012-2015, D. Stuerga intervenes under the same conditions in an ANR-LIMA industrial research project (Light Interactions Materials Aspect, www.pole-moveo.org/pdf-projets-das/Lima-A. pdf).). This project associates 6 partners including the Ecole des Mines de Paris and the PSA group for an amount of 2 MEuros. This project aims to develop model nanometric pigments for goniochromatic paints. Finally, a study contract with Naxagoras Technology as co-financier (MicroNano 3, SMT6-ONOV 2012, 20 KE) will allow testing of a new version of reactor in 2013. [/kc_column_text][/kc_column][kc_column width="20%" _id="361058"][/kc_column][/kc_row]